Executive Summary
Cytochrome c Oxidase (CcO) is the terminal electron acceptor in the electron transport chain. It catalyzes the reduction of dioxygen to water, a process involving the addition of four electrons and four protons. This reduction is also coupled to the pumping of four protons across the mitochondrial inner membrane, which assists in the generation of the proton gradient required for ATP synthesis.
The catalytic mechanism of CcO has yet to be resolved, but several mechanisms have been proposed. Synthetic model compounds are also used to try to replicate the catalytic process of this enzyme. The pumping of protons through CcO is also an immensely complex process that has yet to be mechanistically resolved. Regardless, the data currently point towards a reasonable route of unidirectional pumping deemed the H-pathway.
Contents
1 Context and Structure
1.1 Context in the Electron Transport Chain
1.2 Structure
1.3 Metal Centers and Electron Transfer
2 Catalytic Cycle
2.1 Literature Example
2.2 Controversy
3 Proton Pumping
3.1 Overall Mechanism
3.2 H-Pathway
1.1 Context in the Electron Transport Chain
Cytochrome c Oxidase (Complex IV, CcO) is the terminal electron acceptor in the electron transport chain (ETC). The ETC is located in the inner mitochondrial membrane of eukaryotic cells and the plasma membrane of prokaryotic cells [Bertini]. The ETC couples the redox transfer of electrons to the generation of a proton gradient. In eukaryotic cells, protons are pumped from the mitochondrial matrix (encapsulated by the inner membrane), to the intermembrane space. The electrochemical potential generated by the proton gradient powers the generation of ATP by ATP synthase (Complex V) [Bertini].
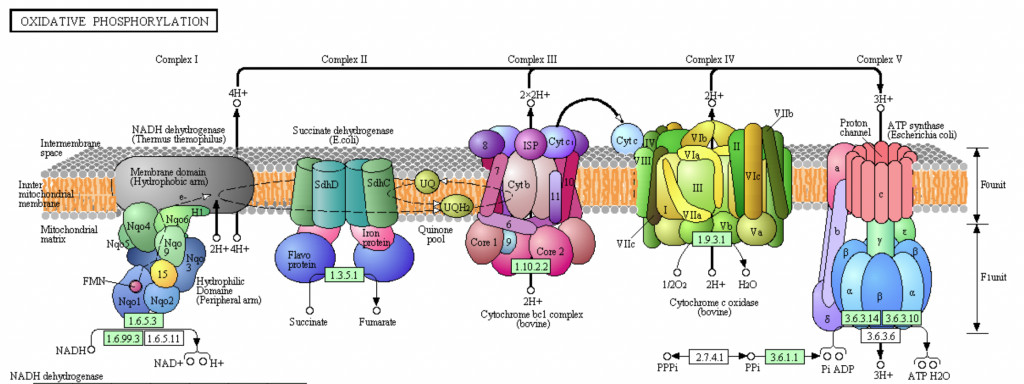
Fig. Overview of the electron transport chain. Cytochrome C Oxidase (Complex IV) is depicted in green, adjacent to ATP synthase (Complex V) [KEGG PHOTO].
Cytochrome c Oxidase reduces dioxygen (O2) to water [Bertini]. This requires four electrons and four protons. Electrons are donated from the electron carrier cytochrome c and the four protons are transferred from the matrix via several pathways. Each cytochrome c only carries one electron, thus four cytochrome c molecules must be reduced to complete the reaction. In the process of dioxygen reduction, CcO also pumps four protons across the inner membrane. The net reaction is as follows:
4Cyt cred + 4H+ + O2 + 4H+matrix → 4Cyt cox + 2H2O + 4H+intramembrane space
1.2 Structure
The structure of bovine CcO was first determined in 1995 [Bertini]. This was a major breakthrough in scientific understanding of this enzyme. Membrane proteins are notoriously challenging to crystallize, and CcO is particularly large (approx. 400 kDa as a dimer), further complicating its crystallization [PDB]. The figure below shows a surface structure of bovine CcO embed in the mitochondrial membrane:
The eukaryotic structure is composed of 13 subunits. The three largest subunits are encoded by mitochondrial DNA and form a reactive enzyme core. These subunits are conserved across prokaryotes and eukaryotes and are responsible for the majority of catalytic activity. The remaining 10 subunits are encoded by nuclear DNA and are not as well characterized. In the figure below, they are shown in green and blue colors:
1.3 Metal Centers and Electron Transfer
There are four key redox active metal centers in CcO. The location of these centers can be visualized in the figure above.
The first of these is the CuA site. The CuA site is a binuclear copper center. Two two Cys thiolates bridge two Cu ions forming an M2S2 rhombohedral structure. The tetrahedral coordination of the Cu ions is completed by neighboring residues. The CuA site is the first redox site to receive electrons donated by cytochrome c. Donation of an electron reduces the site from its oxidized form: CuI/CuII –> CuII/CuII
Upon reduction, the CuA site quickly falls back to the oxidized state, releasing an electron through a 19 angstrom path to the Heme a site.
CcO contains two redox active heme A cofactors. Heme A is distinguished from other hemes by a hydroxyfarnestlethyl group (long carbon chain at the top left) and a formyl group (bottom left). Within the CcO structure, Heme a is coordinated by two histidine imidazole groups, while Heme a3 is coordinated by only a single histidine imidazole. Electrons are received by Heme a and passed to Heme a3, a 14 angstrom distance.
The CuB site is the final redox active metal site in CcO. It is coordinated by three histidine imidazoles, one of which is posttranslationally ligated to a nearby tyrosine residue (Tyr244). This ligation is mechanistically relevant, as it allows for electron transfer from CuB to Tyr244.
Heme a3 and CuB collectively make up the catalytic site of dioxygen binding and reduction. This site can also bind respiratory inhibitors, such as CO, CN–, and N3–.
2 Catalytic Cycle
The catalytic cycle of CcO is still debated. Many of the intermediates are formed at rates that require faster spectroscopic methods than those that are available today [Bertini]. Despite this fact, many intermediates can be observed and have been used to postulate a full catalytic mechanism.
2.1 Literature Example
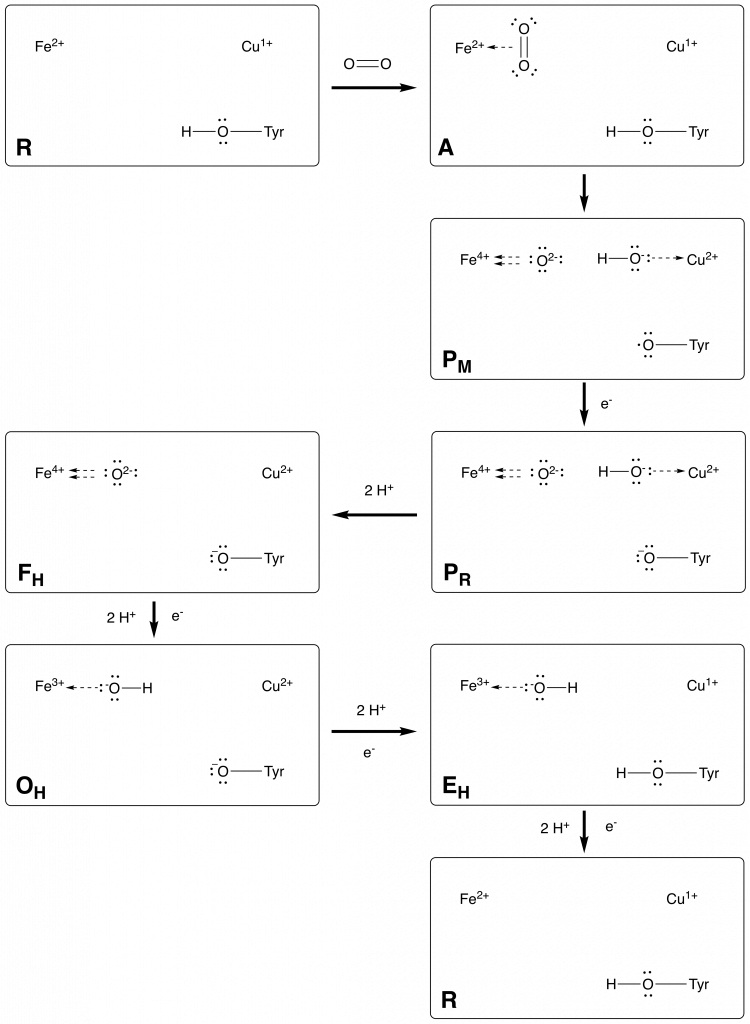
Fig. Catalytic cycle proposed in source [Catalytic Cycle]
3 Proton Pumping
3.1 Overall Mechanism
As evident in the net reaction equation, Cytochrome c oxidase consumes 4 protons upon the reduction of O 2 and translocates 4 protons from the matrix space to the intramembrane space.
4Cyt cred + 4H+ + O2 + 4H+matrix → 4Cyt cox + 2H2O + 4H+intramembrane space
While the two processes are mechanistically linked, the proton channels are distinct. Figure 5 depicts the K-Pathway, D-Pathway and H-Pathway. The K- and D- pathways, named for residues K319 and D91 respectively, are responsible for the protons pumped to the O 2 reduction active site. Both pathways form hydrogen-bond networks to shuttle protons to the active site. The H-Pathway, named for its proximity to Heme a, has a more complicated mechanism of action and is responsible for pumping protons across the mitochondrial membrane, contributing to the electrochemical gradient that powers ATPase.
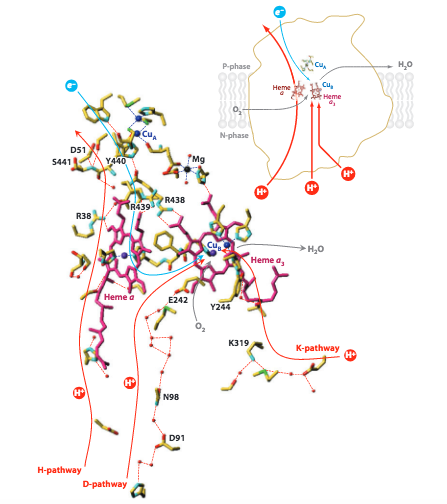
Figure 5. Schematic image of the three proton pumping pathways of Cytochrome c oxidase. Image from Yoshikawa, S. (2011).
3.2 H-Pathway
The scientific community is not in absolute agreement about the mechanism by which protons are translocated across the mitochondrial membrane in CcO. However, current literature points to both the reasonability and energetic feasibility of the proposed Pathway-H (Figure 6).
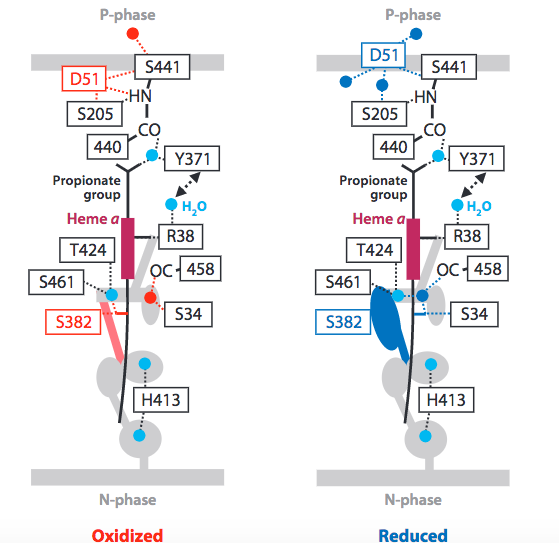
Figure 6. Current proposed mechanism of proton translocation, via H-Pathway. Image from Yoshikawa, S. et al. (2011).
The pathway begins with residue R38, which is accessible via a water channel to the matrix space (N-phase). This accessibility has led researchers to term R38, “protonically labeled”, indicating that the residue is in equilibrium with the matrix space. Next, protons are shuttled past Heme a via an electrostatic repulsion generated by the increasingly positive redox state adopted by Heme a after it donates an electron to O 2 reduction (1.3 Metal Centers and Electron Transfer). In addition, this electrostatic repulsion serves to link the two mechanistic processes (dioxygen reduction and proton pumping) and preserve a 1:1, electron:proton ratio. Next, upward proton movement is aided by an energetically favorable keto to enol transition of the Y440 and S441 peptide linkage. Translocation is completed by D51 which acts as a “swing arm” to transfer protons to the intramembrane space (P-phase).
Reverse proton transfer is prevented by conformational changes to CcO triggered by the complete reduction of O 2 . Burying of the D51 residue within the membrane and narrowing of the lower water channel are two ways in which CcO ensures unidirectional, coupled proton transfer.
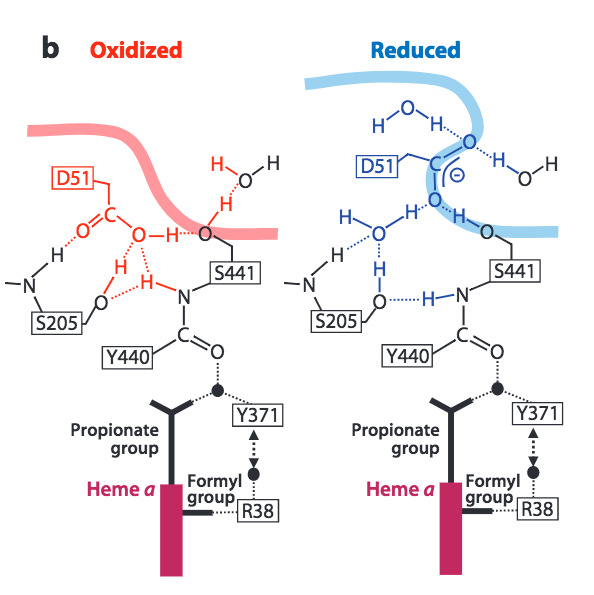
Figure 7. Conformational changes to CcO in the upper H-Pathway linked to the reduction of dioxygen to water. Image from Yoshikawa, S. et al. (2011).
Sources
[Catalytic Cycle] Sharma, V.; Karlin, K.; Wilkstrom, M. “Computational study of the activated OH state in the catalytic mechanism of cytochrome c oxidase.” PNAS. 2013, 110(42), 16844-16849.
[Bertini] Yoshikawa, S. XI.6. Reducing Dioxygen to Water: Cytochrome c Oxidase in Biological Inorganic Chemistry: Structure and Reactivity (eds. Gray, H.B.; Stiefel, E.I.; Valentine, J.S and Bertini, I.). University Science Book , 2006.
[PDB] Goodsell, D. “Cytochrome c Oxidase”. PDB Molecule of the Month. 2000. https://pdb101.rcsb.org/motm/5
[KEGG PHOTO] http://www.genome.jp/kegg-bin/show_pathway?hsa00190
[Proton] Yoshikawa, S., Murmamuto, K., Shinzawa-Itoh, K. Proton-Pumping Mechanism of Cytochrome c Oxidase. Annu. Rev. Biophys. 2011 , 40, 205-223.